Periods of famine are a fact of life for many animal species, including our own ancestors. The resulting natural selection has favored the tendency to set aside energy reserves in the form of body fat, which can be drawn upon when food becomes scarce. Furthermore, when faced with nutrient shortages, many animals shut down reproduction (an example being undernutrition-induced amenorrhea in humans), an adaptive strategy that maximizes lifetime Darwinian fitness by promoting immediate survival and the chance to breed when food again becomes available. However, these options are not available to juveniles, which in most species cannot suspend their development and wait out the difficult times. Rather, they must continue their development and growth, processes limited by the availability of protein which the body cannot store as it does with fat. Thus, undernutrition at a juvenile stage typically results in stunting and has lifelong health and fitness consequences.

Can animal physiology evolve in ways that would help juveniles to grow and develop under chronic nutrient shortage? What changes in the use of the scarce nutrients would this involve? To find out, we bred genetically variable populations of fruit flies (Drosophila melanogaster) on a nutrient-poor larval diet for more than 240 generations. When confronted with the poor diet for the first time, Drosophila larvae take twice as long to develop as they do on standard lab diet, yet the resulting adults barely attain half of the normal body weight. In the course of evolution in our experiment, populations became more tolerant to the poor diet, attaining faster larval growth and cutting the developmental delay by half. They achieved this tolerance in part by becoming more efficient at extracting and absorbing scarce amino acids from the poor diet. Yet, when we examined their larval metabolome (the abundance of diverse metabolites), we found that they harbor lower levels of many amino acids in their system, compared to control larvae experiencing the poor diet for the first time. This is particularly the case for the branched chain amino acids (leucine, valine and isoleucine), a trio of structurally related essential amino acids that together account for about 20% of protein content. The levels of free amino acids are supposed to reflect the nutritional status of the organism; in particular, low levels of leucine are thought to signal nutritional deprivation, leading to inhibition of protein synthesis and growth. Yet our malnutrition-adapted larvae are nutritionally better off and grow faster on the poor diet than non-adapted controls. So, what is going on?
To gain some understanding, one should first note that the free amino acids do not in themselves play a major functional role – their principal use is as building blocks in protein synthesis, fueling organismal growth. Thus, their reduced concentration in malnutrition-adapted larvae, despite faster absorption from poor diet and faster larval growth, is suggestive of more efficient cellular logistics, whereby raw materials for growth are used as soon as they are available. By analogy, stacks of building material laying around a construction site suggest poor organization or labor bottlenecks rather than a high speed of construction; an efficient company will make sure that materials are delivered when needed and used immediately.
However, amino acids can also be used as a source of energy. The first step in this process is deamination, i.e., removal the NH3 group for ultimate excretion as a waste product (in the form of urea in mammals, uric acid in insects, birds and reptiles). The remaining carboxylic skeleton can then be directly used to generate ATP (the cellular energy carrier) or converted to glycogen or fat as energy storage. Obviously, larvae need energy for growth, foraging and other life-sustaining processes, and they must also store some fat to fuel the energetically costly process of metamorphosis. “Burning” the spare amino acids, however, seems wasteful, especially given that we have shown that larval growth on poor diet is limited by dietary protein rather than carbohydrates.
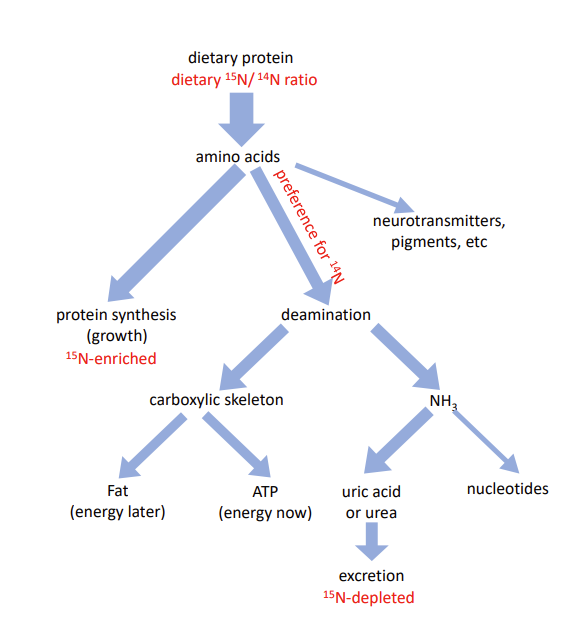
We therefore hypothesized that the malnutrition-adapted larvae would minimize “burning” of amino acids and instead derive all their energy from dietary carbohydrates (from cornmeal and sugar contained in the food medium). To test this hypothesis, we exploited the fact that the deamination reactions have a slight preference for NH3 groups containing the common 14N isotope. As a consequence, growing larval tissues accumulate the heavier stable isotope 15N in proportion to the fraction of amino acids that are deaminated with their nitrogenous waste product being excreted. Thus, we expected that the malnutrition-adapted larvae would show a lower 15N/14N ratio at the end of their development than larvae from control populations. But we found the opposite: while the 15N/14N ratio in control larvae raised on poor diet was indistinguishable from the ratio in the diet, malnutrition-adapted larvae showed elevated levels of 15N, although not as high as larvae raised on protein-rich diets. This implies that while larvae with no (recent) evolutionary history of undernutrition respond to it by hoarding amino acids, evolutionary adaptation favored a limited use of amino acids as energy source despite their scarcity. This conclusion is corroborated by differences in the levels of the nitrogenous waste product uric acid.
To interpret this unexpected results, it helps to know that although the malnutrition-adapted larvae are better at extracting amino acids from their poor diet, they are less efficient at extracting carbohydrates, an apparent trade-off in acquisition of scarce macronutrients. Thus, not only can they afford to “burn” some amino acids; they may be compelled to do so by not obtaining enough dietary carbohydrates.
One paradox remains unresolved. The rate of protein synthesis in cells is regulated by the TOR signaling pathway based on the levels of free amino acids, in particular leucine. This prevents cells from initiating the synthesis of more protein molecules than it has amino acids to complete. The low level of leucine in the malnutrition-adapted larvae should thus inhibit protein synthesis and organismal growth by suppressing the TOR pathway. This does not seem to happen, suggesting that the evolutionary adaptation to nutrient shortage in our evolution experiment also involved changes in the TOR pathway and/or other mechanisms that regulate cellular growth and division in response to nutritional situation. This is one of the directions we are now exploring.
Our study demonstrates that the metabolism of juvenile animals can rapidly evolve to alleviate consequences of nutrient shortage for growth and development; physiological adaptation to famine is thus not limited to storing fat and shutting down reproduction. It remains to be seen what side effects and trade-offs such metabolic adaptation may entail, but our results boost the idea that aspects of human metabolism that make us vulnerable to disease may have been favored by natural selection in our ancestors during periods of famine.