Dr Domino Joyce explains what her team’s new research tells us about how animals adapt to challenging environments – and how quickly it can happen.
As we explore more of the earth’s extreme environments, we learn more about how
organisms have adapted to life there. Many deep sea creatures have become familiar such as the vampire squid, spookfish or blobfish, and we are beginning to study their biological adaptations to dark, sometimes cold, high pressure environments. But there are also many less well studied freshwater environments that pose similar problems for any organism wishing to inhabit them, and our work studied a group of closely related deep water fish that live in Lake Malawi, in East Africa.
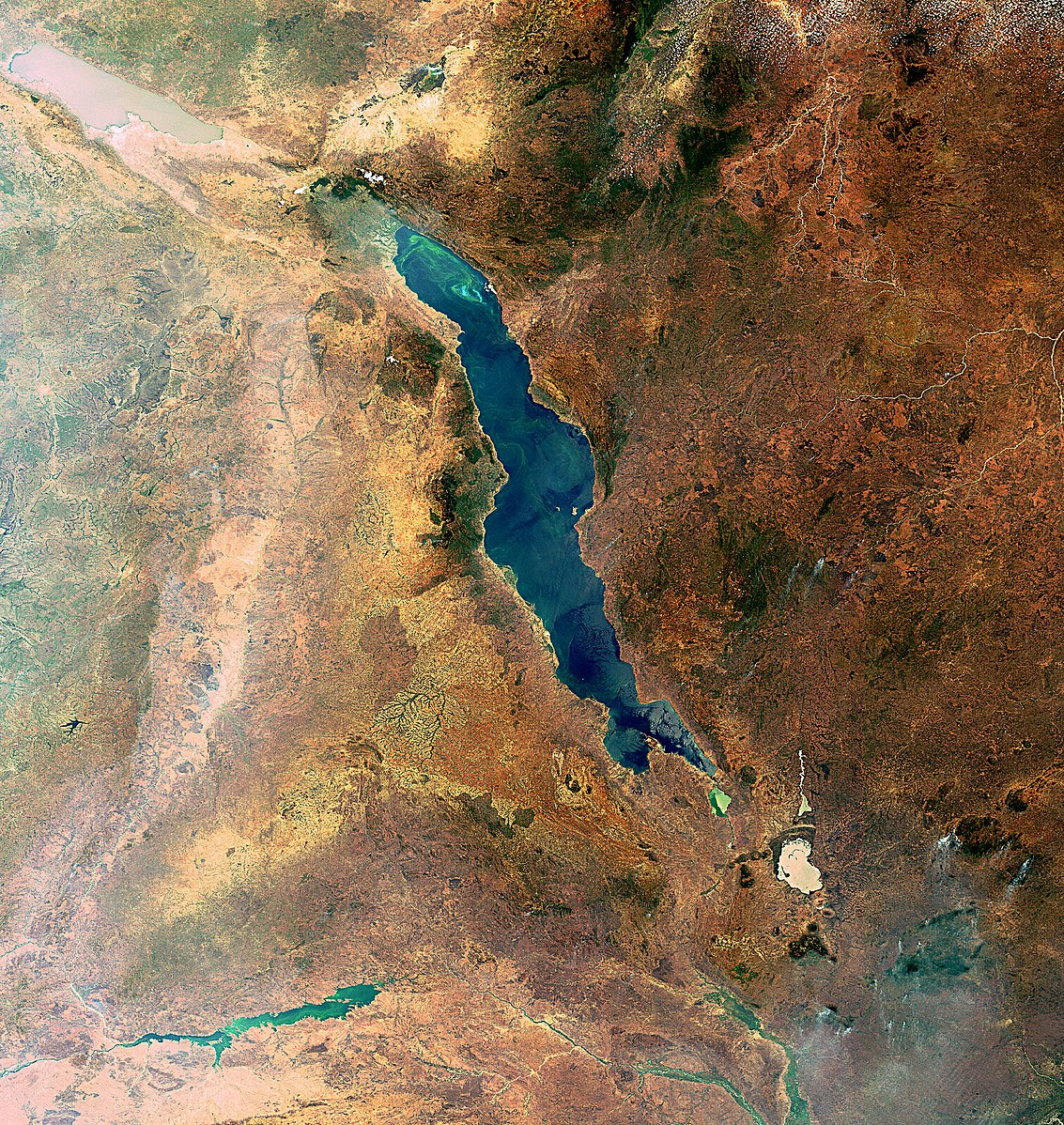
Lake Malawi is home to more than 800 different species of cichlid fishes, which is particularly impressive because they must have evolved in the lake sometime in the last 2–5 million years (they aren’t found anywhere else, and before this time, the lake didn’t exist). These species are found in a range of environments throughout the lake, from shallow-water rocky reefs, where they have body, mouth and tooth shapes that help them scrape algae for nutrition, to the open water, where some species have become large and streamlined predators that chase and eat other fish. We studied four species of a deep water genus called Diplotaxodon, these fish are among the deepest living cichlids, found between 60 and 250 m, and they feed on zooplankton. We sequenced parts of their genome, using a technique called RAD-sequencing, to try to understand how their adaptations to depth have evolved.
Above: Cichlid fish from the deep water genus Diplotaxodon
Positive selection favours traits that increase an individual’s chances of survival and/or
reproduction in a given environment. But environments differ and the traits that are adaptive in one environment may be different elsewhere. Genetic divergence between species normally happens very slowly over evolutionary time, but may be accelerated in genomic regions and genes that control or are involved in the regulation of such adaptive traits. We compared DNA from a number of individuals from each of the four Diplotaxodon species, and found regions in the genome that had diverged from one another very strongly compared to the average genomic divergence, indicating that these regions may contain candidate genes particularly important for the evolution of these species and their survival in their challenging environment.
Among these candidates were genes involved in head and eye development. Some of our deep water species have relatively large eyes, which is thought to be adaptive in deep water environments because larger eyes increase the chances of capturing the minute amounts of light that penetrate the deeper water layers. We therefore think this divergence may be a signature that selection has acted on these genes.
Another divergent region was centered around a gene which codes for a protein in the lens of the eye – phakinin. We think that mutations in the sequence of this gene, which we were able to study thanks to the Sanger Centre, Cambridge, who shared some of their genomic data with us – affect the transparency of the eye lens, so that light can pass the lens more efficiently, thus relatively more light can reach the retina and can be detected by these fish in deep water.
Finally, we found a further divergent region that contained genes coding for subunits of the oxygen-carrying haemoglobin, and this lead us to examine the particular mutations in these genes too. We found consistent differences between variants of these genes occurring in deep- and shallow water species. Mutations in these genes have been shown in other species, including humans, to affect the affinity of the haemoglobin molecules for binding oxygen. Increased oxygen binding affinity is another potentially adaptive trait in deep freshwater environments where the amount of dissolved oxygen is relatively low. Most teleost fish possess a so-called swim bladder, a specialized gas filled organ that allows them to control their buoyancy in the water by actively regulating the gas pressure in the bladder. The greater the water depth, the higher the hydrostatic pressure, the higher the gas pressure in the bladder needs to be to retain neutral buoyancy. Teleosts have evolved a complex physiological system to ‘pump’ oxygen against a pressure gradient from the blood into the bladder. Central to the system is a phenomenon known as the Root effect, which is the drastic reduction of the oxygen carrying capacity in response to slight changes in pH. In teleosts special ‘Root haemoglobins’ transport and locally release the oxygen used for inflating the swim bladder. The molecular basis of the Root effect isn’t well understood yet, but we think that the mutations that we observe in the deep water species may facilitate the production of the high gas pressures that would be required in the deep.
Finding the parts of the genome that have helped these fishes colonise and adapt to the depths of Lake Malawi is interesting in itself, but it also demonstrates that at least some of the diversity in Lake Malawi cichlids is a result of “de novo” mutations that have arisen in the lake and then been increased by selection once proven advantageous. That this can happen in such a short evolutionary time frame is impressive because we usually associate such changes with a much longer timespan. But our work may also provide an insight into adaptations in other living systems- for example we would predict that the lens clarity gene that has differentiated in these species might have evolved in the same or similar ways independently in large eyed deep water marine fish. We might find important mutations at the same points in the haemoglobin coding genes of animals that have adapted in different ways to other challenging environments, such as high altitude.
Identifying the possible mutational changes in the DNA that lead to adaptations to extreme environments may help our understanding of particular physiological processes, but also of evolution in general. Finding these examples in Lake Malawi cichlids means that we also now know that this type of adaptation can arise surprisingly quickly.
To learn more, read Dr Joyce’s paper, published open access in Evolution Letters.