A new study published in Evolution Letters uses an experimental approach to provide new insights into the evolution of parasite resistance across populations. Author Dr. Amanda Hund tells us more.
Parasites and pathogens are a common and powerful force in the natural world, a fact many of us know all too well in the middle of a global pandemic. It has long intrigued biologists that some hosts are able to resist parasite infections while others remain highly susceptible. Even among closely related populations of the same species, the ability to fight off a parasite often varies dramatically. Because parasites can reduce survival and reproduction, this can have important evolutionary consequences. Yet, our understanding of the details of how parasite resistance evolves is still limited. To tackle this important question, it is useful to think about the interaction between a host and a parasite as a series of sequential steps. First, the host must encounter a parasite (exposure). Next the host must recognize that it is infected and initiate the right immune response. Then, the host must be able to carry out that response to a high enough level to kill or at least slow down the parasite. Finally, because immune responses are costly, once the host has cleared an infection it must be able to turn off that response. Determining where resistant and susceptible populations differ in that timeseries of events can help us figure out where evolution is occurring between hosts and parasites.
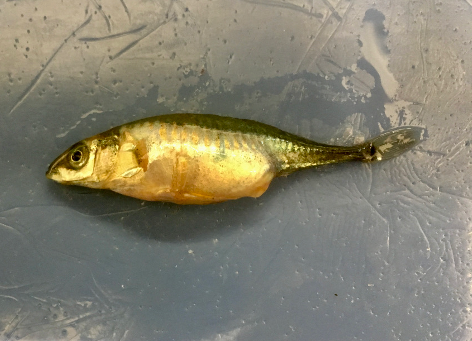
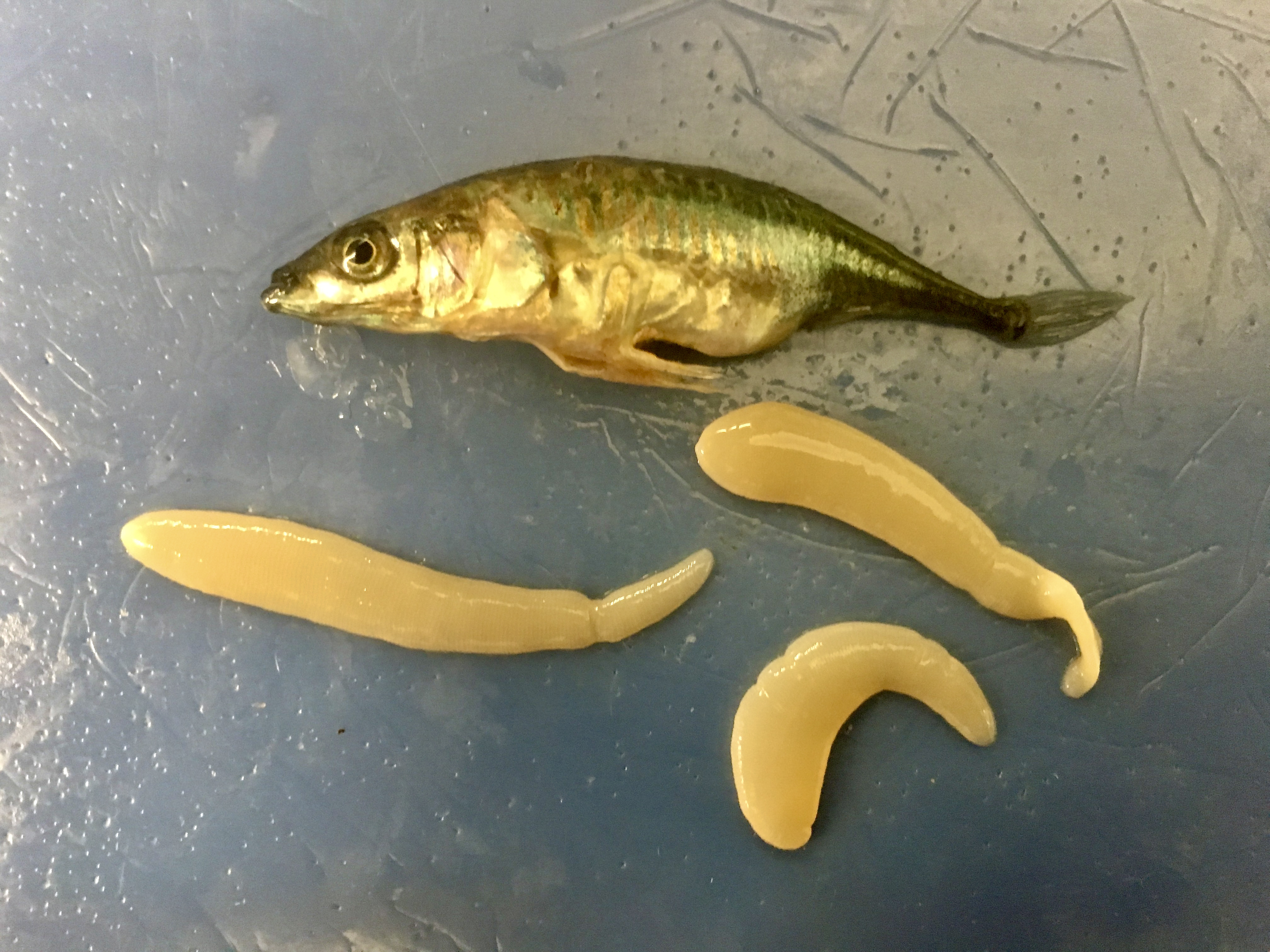
Around 11,000 years ago, marine dwelling threespine stickleback fish took advantage of newly uncovered lakes as the Pleistocene glaciers melted along the west coast of Canada on Vancouver Island. While these lakes turned out to be a pretty good place to live, the fish were faced with a dangerous new parasite- a freshwater tapeworm that infects the abdomen of the fish and can grow to be over half its body weight. When full grown, this tapeworm can make it difficult to swim or reproduce and even manipulates the fish’s behavior to increase the odds that it will be eaten by a bird, where the tapeworm reproduces. Today, some lake populations of stickleback are still heavily infected with large tapeworms, but others have evolved the ability to resistant the tapeworm by encasing it in fibrous scar tissue, called fibrosis. These fish can prevent the tapeworm from growing and sometimes even kill it by trapping it in fibrosis. This is particularly interesting because fibrosis also occurs in humans, where it contributes to a wide variety of diseases. The question of how fibrosis stimulation, severity, and recovery, may evolve is thus both fundamental to our understanding evolutionary diversification, and may have applied benefits to understand human disease as well.
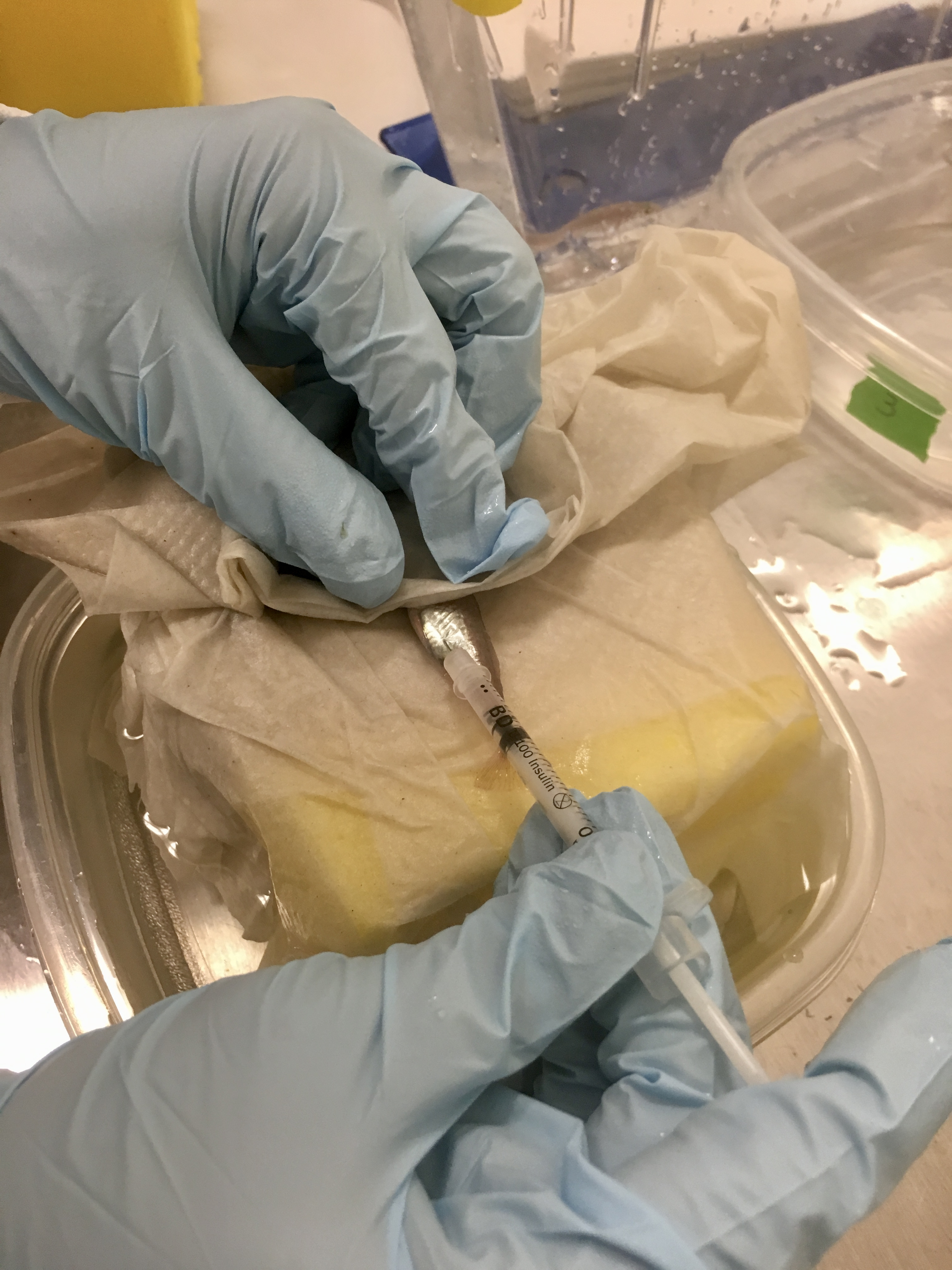
We compared a resistant lake population, a susceptible lake population, and an ancestral marine population of fish to figure out how this resistance has evolved. We used an experiment to tease apart the different steps in the host response to the tapeworm to figure out how these populations differ from one another. We injected four different immune challenges into the abdomen of fish and tracked their fibrosis response through time. These included 1) a saline control, 2) alum, which is a general immune stimulant commonly used in vaccines, 3) tapeworm protein which we extracted from ground up tapeworms, and 4) a combination of alum and tapeworm protein. We did this experiment in lab raised fish from each population, to ensure that any differences we found were due to evolution and not just because of ecological variation between lakes.
We found that with enough time, all three populations were able to mount a strong fibrosis response to the alum treatments, meaning their ability to produce fibrosis was universal. However, only the resistant population was able to produce fibrosis to the tapeworm protein on its own. This indicates that the resistant population was able to recognize a tapeworm infection and initiate fibrosis, while the other populations could not. We also found that the resistant population was able to start producing fibrosis much faster than the other populations, within 24 hours after the injection. Evolution has likely favored this fast reaction so that these fish can control and kill tapeworms while they are small. Towards the end of our experiment, we also discovered that the resistant population was able to start clearing their fibrosis, while the other populations continued to have lots of fibrosis from the alum treatments, even up to 90 days after the injection. Other studies have found that fibrosis can be costly for stickleback, decreasing their ability to swim and reproduce. To help avoid these costs, resistant fish appear to have evolved the ability to resolve fibrosis after clearing a tapeworm infection.
So, at what step does parasite resistance evolve? According to our results, the critical point for our stickleback populations is when fish first detect the parasite and initiation their response. Our study also demonstrates that success or failure at each step in the host response influences whether evolution will be able to act on the following steps. Because resistant fish can recognize and respond to tapeworms, they have evolved to respond rapidly, while tapeworms are small, and resolve that response once the danger has passed. By experimentally isolating different stages of the host response to infection, we were able to discover where key differences lie between resistant and susceptible populations.
Dr Amanda Hund is a James S McDonnell Foundation Postdoctoral Fellow at the University of Minnesota. The original article is freely available to read and download from Evolution Letters.