A new study in Evolution Letters has investigated the genetic basis of long life in the fruit fly Drosophila melanogaster. We quizzed the leader of the research, Professor Thomas Flatt, on his group’s findings and what they mean for our understanding of ageing.
EL: Your study set out to identify the mechanisms by which longer life evolves. What were your expectations going into this research?
TF: Since the 1980s a lot has been learned about the genes and the molecular pathways that affect ageing and longevity, most prominently genes involved in insulin signalling, but also in other pathways. We therefore had the clear – but in hindsight, naïve – expectation that we would find pronounced evolved genetic changes in some of the key pathways that had been discovered in mutant screens and transgenic analyses. While it was clear that we would not identify the kind of (typically deleterious) large-effect mutations that mutant screens have uncovered, we expected to find more subtle genetic changes in some of these well recognised ‘longevity’ genes and pathways. When I started planning this project with my colleagues my vision was that this experiment would allow us to learn more about the role of natural, standing genetic variation in insulin signalling and how it affects lifespan. Although we did find some changes in a few well-known genes and pathways associated with insulin signalling, we were rather surprised to find a lot of changes in genes whose link to lifespan was unknown. Perhaps most surprisingly, we found an enrichment of evolved changes in immune genes. While it intuitively makes sense that immune function is involved in ageing, and while a lot of current mechanistic research is studying ‘immuno-senescence’ and ‘inflamm-aging’, we were nonetheless intrigued that our analyses turned up immunity genes as one of the ‘top’ mechanisms underlying evolutionary changes in lifespan: these immune genes are not traditionally known to play an important role in lifespan. Since the 2000s there have been a few papers here and there suggesting that immune genes in flies can affect lifespan, but for the most part the connection between lifespan and immunity remains poorly understood to this day. Our observation, combined with reading papers about similar lifespan selection experiments by Kim Hughes’ lab at Florida State and Trudy Mackay’s group at North Carolina State, prompted us to explore the role of immunity in lifespan.
EL: You used lines of Drosophila selected for late-life fertility. Can you tell us more about these selection lines, and why they were ideal for testing your ideas?
TF: The lines we used were first published in 1984 in a now famous paper by Leo Luckinbill, our coauthor Bob Arking, and colleagues. At the same time Michael Rose published a back-to-back paper in Evolution on an independent but qualitatively similar selection experiment. These two papers were ground-breaking – they showed for the first time that lifespan can be made to evolve in the laboratory. To study the genetics underlying the evolution of lifespan we had to rely on such ‘laboratory evolution’ lines rather than long-lived mutants from mutant screens, because large-effect mutants are very unlikely to reflect the sort of genetic variants that are segregating in natural populations i.e. the substrate of evolutionary change. The problem is that in many organisms it is very hard (and time-consuming!) to produce long-lived individuals via selective breeding – this is most easily done in relatively short-lived organisms. The fruit fly is optimal for this purpose. The advantage of the lines we used is that they have been maintained under selection for over 35 years, thus allowing sufficient time for profound evolutionary genetic changes to be detectable with genome sequencing. In shorter selection experiments, detecting adaptive genetic changes is often a challenge because the evolved genomic patterns may not be that clear-cut yet.
EL: What techniques did you use to unravel the genetic mechanisms at play?
TF: The technical work involved was fun but also a lot of hard work, especially for two first authors on the paper, Daniel Fabian and Kathrin Garschall, two former PhD students. Although we already had the evolved flies (from Bob Arking), so did not have to perform a laboratory evolution experiment from scratch, we had to use a wide array of methods to nail down the genetic mechanisms: next-generation sequencing; bioinformatics, statistics, and combinatorics; infection assays with different pathogens; measurements of gene expression; transgenic constructs (RNAi) to knock down the expression of candidate genes; and finally assays to measure the lifespan of flies. Our ambition was not to just perform a population genomic analysis of the selection lines, which would have been quite quick, but also to examine and validate some of the physiological and genetic mechanisms that we discovered via genomics. To make things even more challenging, my lab moved three times throughout the course of this work: from Vienna to Lausanne to Fribourg – in the end it took us almost 7 years to complete this project.
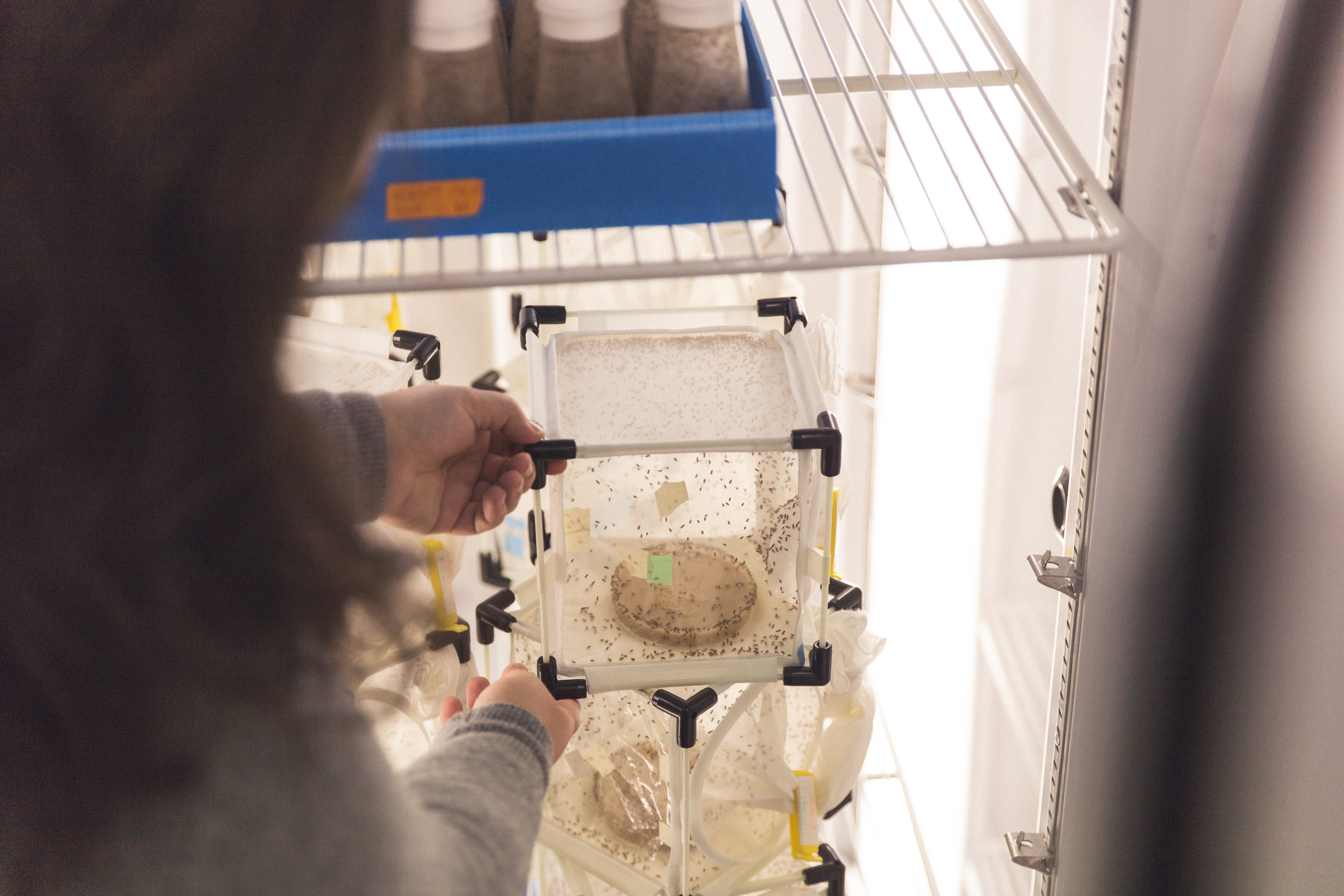
EL: A key finding of your work was that the evolution long life is underpinned by the evolution of immunity. Why do you think these two things are linked?
TF: Based on our observations, I think that the long-lived flies we have studied might have evolved an improved age-dependent regulation of their immune system, a regulation that allows them to fight off infections better while at the same time reducing the costs associated with deploying the immune system. We were intrigued to see that at old age, short-lived flies up-regulate immune effectors but are worse at fighting off infections, whereas old long-lived flies tend to down-regulate these effectors, yet are better capable of fighting infections. This suggests to us that long-lived flies have evolved reduced immuno-senescence and might suffer less from immunopathology. Thus, we strongly believe that the evolution of an improved immune system contributes to the evolution of long life. To what extent this might be a general phenomenon remains to be seen.
EL: How comparable do you think the genetic mechanisms you’ve identified in Drosophila will be to those in other animals, particularly those with longer lifespans such as large vertebrates?
TF: A prime example is the insulin signalling pathway mentioned above: this pathway seems to have evolutionarily conserved effects on ageing and lifespan across many organisms, from flies and nematode worms to humans. From work in the nematode worm C. elegans we know that long-lived worm mutants in the insulin signalling pathway are more resistant to pathogenic infections. Similarly, in people who live to over 100 years, certain gene variants in the insulin signalling pathway have been associated with exceptional longevity. Yet, we did not really find a lot of changes in this pathway, as mentioned above – instead we found lots of changes in immune genes. With regard to these immune mechanisms, there are at least three arguments that make me believe that humans and flies might not be so different from each other. In humans it is clear from longitudinal studies that the ageing process often leads to a chronic pro-inflammatory state and that this can contribute significantly to multi-morbidity, disability, frailty and death, This is somewhat similar to aged and frail flies, which have problems fighting off infections, suffer from immunosenescence, and show signs consistent with chronic inflammation. Secondly, there is evidence that healthy, long-lived people have improved anti-inflammatory responses – something similar might explain why the long-lived flies we have studied are better capable of dealing with infections when they are old. Third, the pathway we have studied in our paper – Toll signalling – plays an evolutionarily conserved role in immunity across species: one of our coauthors (Bruno Lemaitre) was the first to discover the role of this pathway in immunity in flies and subsequent work then showed that Toll signalling also plays a role in vertebrate immunity. So I do think that some of the insights gained in flies might – at least to some extent – be applicable to other systems, potentially even to humans. But obviously much more work needs to be done before we can draw general conclusions.
EL: What would you say are the big unanswered questions in this field? Where do you see your own work heading next?
TF: First, we do not know yet whether our observations are general, so more studies in other organisms are needed. Second, the molecular mechanisms underlying our observations still need to be worked out in detail – how can down-regulation of immune effectors at old age actually improve realised immunity and lifespan? How does down-regulation of immune genes affect lifespan mechanistically, i.e. what other pathways and interactions are involved in these effects? How does this regulation work in different tissues across the body? Is there a direct connection between fertility and immunity? At the moment, we are analysing genome-wide gene expression data in the long-lived fly lines to better understand the physiological changes in these flies. But we are working on a lot of other stuff too. We are studying chromosomal inversions and ‘supergenes’ and how these affect fitness traits, as well as performing a comprehensive analysis of genetic variation in flies on the European continent (where flies arrived first 10–15 k years ago when they migrated out of Africa) with an international team of colleagues (the DrosEU consortium). We are also performing transcriptomic analyses of the trade-off between reproduction and somatic maintenance. So we are not going to be bored for a long time!
Thomas Flatt is Professor of Evolutionary Biology at the Department of Biology, University of Fribourg, Switzerland. The original study is freely available to read and download here.