Recent evidence suggests that if we replayed evolution from the same starting point, in the same environment, it would proceed in a predictable manner, with certain genes being repeatedly selected. But what about in a more realistic world, where environments differ? Dr Caroline Turner provides new insights from her experimental study published today in Evolution Letters.
A recent post on this blog, “Testing the Predictability of Evolution”, nicely introduced the captivating subject of the repeatability of evolution. Namely, if we were able to turn back time and evolve the same organisms under the same conditions, would evolution occur in the same way? In their recent paper in Evolution Letters, Härer et al. considered a variant of this question – if similar, but not identical, organisms evolve under the same conditions, will those different organisms adapt in the same way? Our paper, now published in Evolution Letters, asks the complementary question: If identical organisms evolve in similar, but not identical environments, will those organisms adapt to common selection pressures in the same way?
To address this question, we experimentally evolved bacteria in the laboratory. Because bacteria reproduce so quickly and have large population sizes, it is practical to watch how they evolve in a relatively short amount of time in the laboratory. Experimental evolution in the laboratory allows us to evolve many replicate populations all founded from the same ancestor. Each replicate population is then an independent repetition of evolution. Evolution in the laboratory allows us to control the evolutionary environments to a much greater degree than is possible in nature, making sure that the replicate populations evolve in as consistent of an environment as possible. The other benefit of working with bacteria is that we can easily freeze the populations and later revive them from the frozen stocks. This means that we can directly compare the characteristics of evolved populations and their ancestors.
In our experiment, we wanted to test the degree to which our bacterium, the Gram-negative opportunist Burkholderia cenocepacia, adapted in the same way to a given selection pressure, even when another aspect of their environment varied. We varied two aspects of the environment. First, we varied the amount of food (sugar) that was available to the bacteria. We also varied whether selection favored bacteria which grew in liquid (planktonic growth) or on surfaces (biofilm growth). The treatments are summarized below (the second high-carbon, biofilm treatment is a control for the effects of population size):
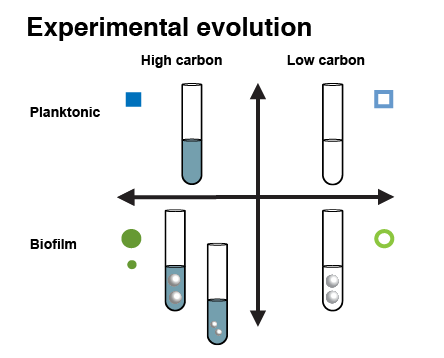
We evolved six replicate populations in each environment for 90 days. As the bacteria replicate in each environment, new mutations arise by chance due to errors in copying DNA. If the bacteria with a particular mutation were able to compete more successfully in their environment, then those bacteria would be able to produce more offspring than bacteria without the mutation. As a result, that mutation would reach a higher frequency in the population over time.
After 90 days of evolution, we sequenced the genomes of the evolved populations to find the mutations that spread in each population. We measured the genetic similarity between populations evolving in each environment. Overall, populations that evolved under a shared selection pressure (either sugar level or mode-of-growth) were more genetically similar to each other than populations that evolved in environments which shared neither selection pressure. For example, populations evolved in a high-sugar environment repeatedly evolved mutations in a protein affecting iron storage, regardless of whether they were selected for planktonic or biofilm growth. These results indicate that evolutionary change can be repeatable, even when environments are not exactly the same.
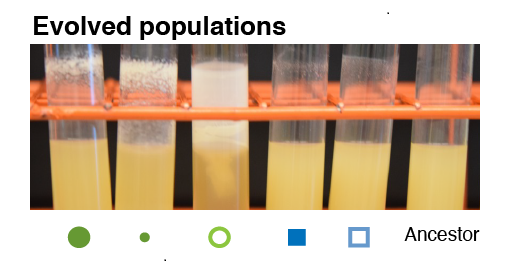
However, there was one exception to the pattern of higher genetic similarity between populations that evolved in similar environments. Populations evolved in low-carbon, biofilm environments had almost no genetic similarity with populations evolved in low-carbon, planktonic environments. The bacteria adapted to low-carbon conditions differently, depending on whether they were in an environment selecting for planktonic or biofilm growth. We think this exception results from a deep tradeoff between investing in rapid growth or synthesizing polymers to produce a biofilm. Such a tradeoff may be masked when resources are abundant but it emerges when resources are scarce and bacteria are forced to commit to either lifestyle. Despite this exception, overall we observed a great deal of predictability to evolution with many parallel mutations occurring both within treatments and between treatments with shared selection pressures.
Caroline Turner is a postdoctoral associate in the Cooper Lab at the University of Pittsburgh. The study is freely available to read and download from Evolution Letters.