In our latest author blog, Mike Hague tells us how specific genetic mutations lead to large-scale ecological trade-offs in a deadly predator-prey system.
Driving north on Highway 101 in California and my car reeks of garter snakes and camping gear. I’ve just left my field site in Sonoma County, where I study a population of common garter snakes (Thamnophis sirtalis) that are caught in a coevolutionary arms race with their prey, Pacific newts (Taricha spp.). The potent tetrodotoxin found in the skin of the newts is deadly to all other predators, but garter snakes at this site have evolved extreme resistance to the toxin. Tetrodotoxin works by binding into voltage-gated sodium channels in nerves and muscles, blocking the transmission of electrical signals. Snakes in Sonoma County evolved four adjacent mutations to their sodium channels that prevent the neurotoxin from binding, allowing the predators to swallow newts whole with no ill effect.
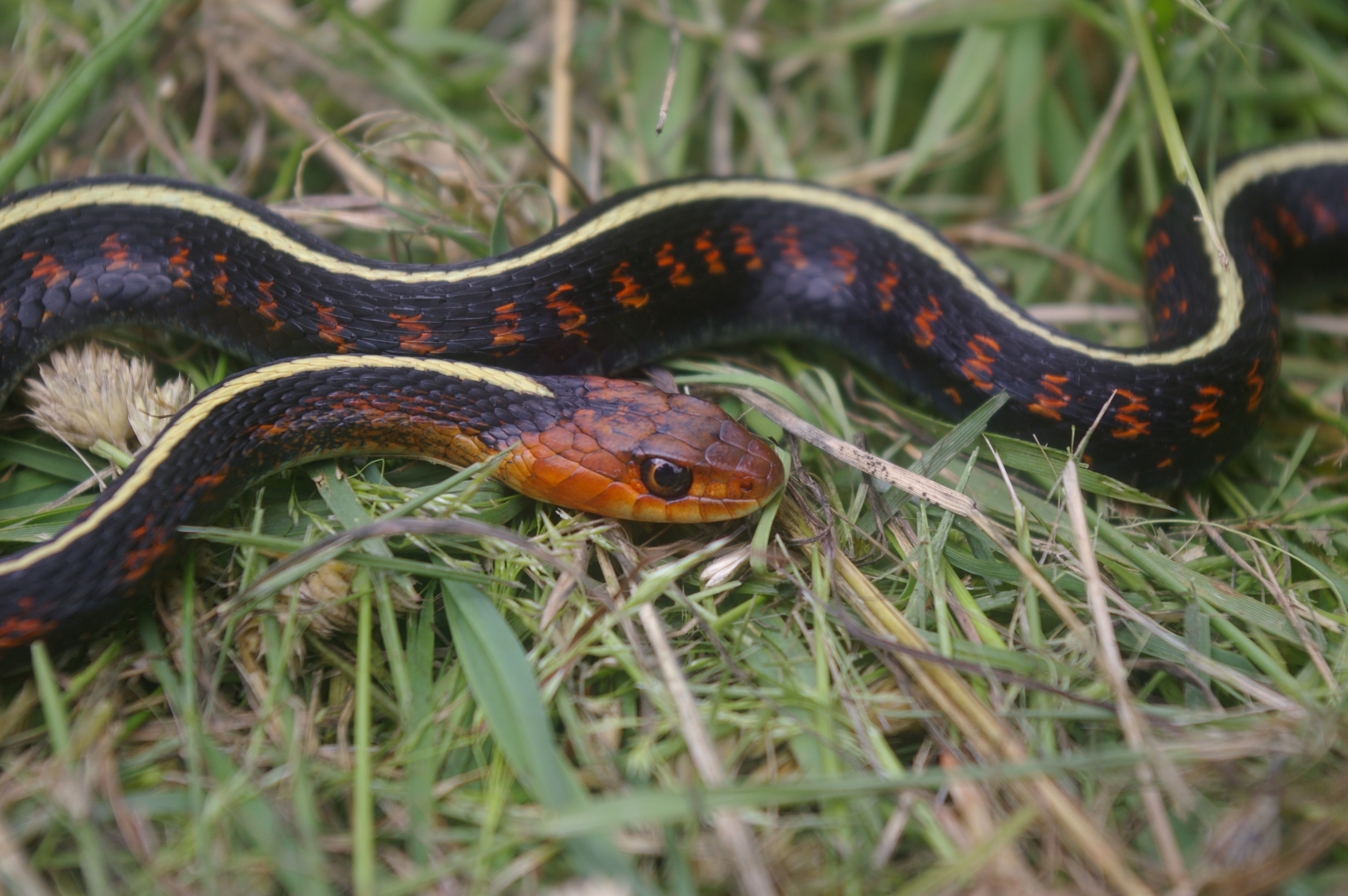
I’m on my way up the Pacific Coast to my next field site in Mendocino County. Garter snakes and newts are found together from central California up the coast to Canada. My next stop is only an hour up the highway—a short drive for a field biologist. Unlike in Sonoma, snakes in Mendocino generally have low levels of resistance to tetrodotoxin and lack mutations to their sodium channels. But why do we see this sharp decline in the level of resistance? Newts are found at both the nearby sites, so shouldn’t beneficial mutations that confer resistance be favored by natural selection in both populations? Perhaps the mutations are disadvantageous at certain sites?
These questions motivated the study published today in Evolution Letters. A persistent question in evolutionary biology is whether the accumulation of beneficial mutations generates trade-offs during the process of adaptation. When populations diverge and adapt to local conditions, compromises can arise between related traits, like virulence and spore production in pathogens or microbial resistance and growth in plants. But whether these trade-offs emerge from the underlying effects of individual genetic mutations is unclear.
The toxin-resistant mutations in garter snakes occur in the sodium channels of muscle tissue, which play a fundamental role in electrical signaling of the nervous system. Because their function is so important, the channels have an evolutionarily conserved structure across all of vertebrates, including humans. As garter snakes evolved greater and greater resistance in the arms race, mutations accrued in the sodium channel to prevent toxin binding. The Sonoma County snakes, with four cumulative mutations, represent the extreme end of escalation in the arms race. We predicted that the accumulation of these mutations would have negative consequences for sodium channel function and muscle performance.
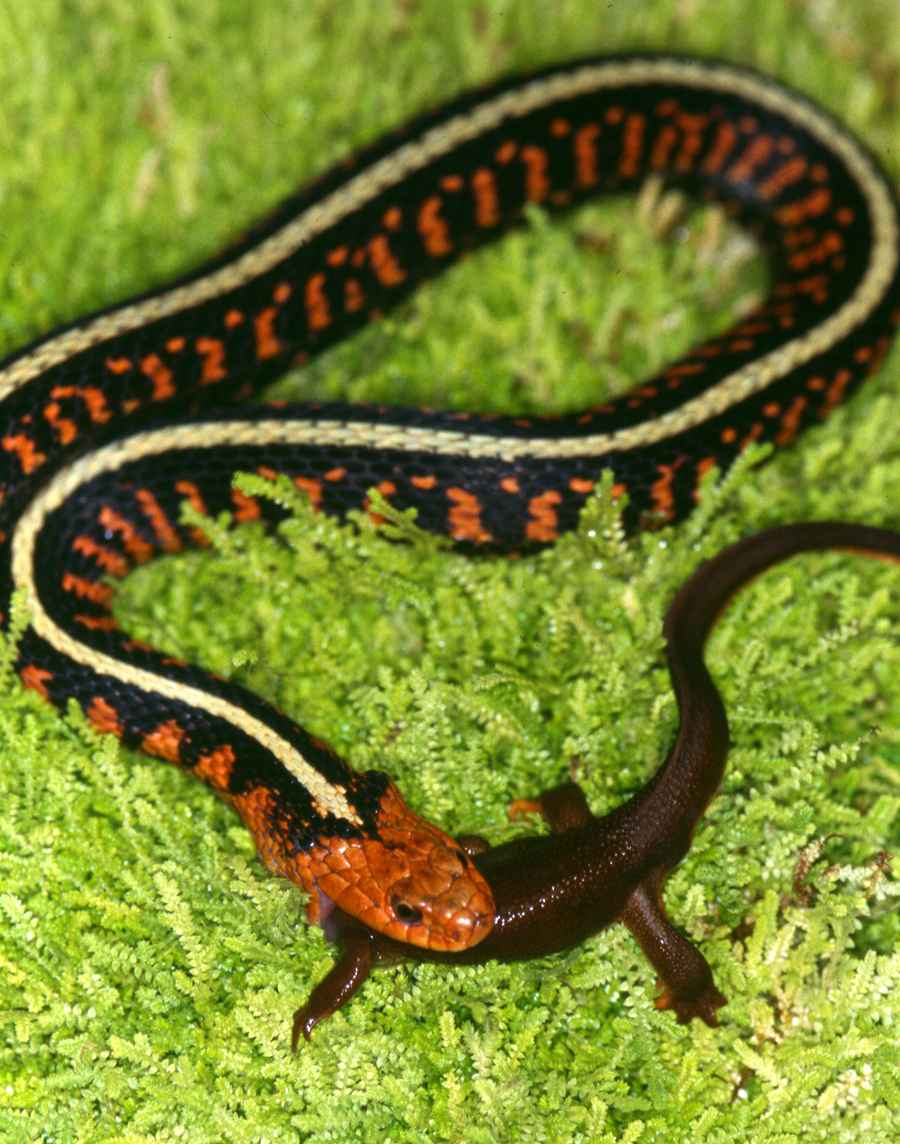
We raced resistant and non-resistant snakes on a racetrack to compare their overall muscle performance and found a clear pattern. Highly resistant snakes with the four Sonoma mutations were significantly slower than the non-resistant snakes that had no changes to their sodium channels. Non-resistant snakes from sites like Mendocino crawled faster, implying the four mutations at Sonoma cause a trade-off between toxin-resistance and muscle performance. We tested other snakes from Oregon and Washington that had intermediate resistance—only one or two mutations to their sodium channels—but they showed no such evidence for a trade-off.
Around the same time, another student in the Brodie lab made a remarkably similar finding. Gabriela Toledo was focusing her studies at the molecular level, testing how toxin-resistant mutations affect the electrophysiological function of sodium channels in the snakes. Training with Shana Geffeney and Charles Hanifin, Gabriela was able to synthesize different snake sodium channels into frog eggs and then record their performance in individual cells. She found that the same four Sonoma mutations that reduce crawl speed also cause diminished excitability in sodium channels. These channels require a higher voltage threshold to initiate electrical signals. Similar reductions in excitability are linked to congenital diseases in humans that cause muscle weakness and paralysis. As in our racetrack experiment, Gabriela only found evidence of this trade-off in the most resistant sodium channels in the arms race, with four cumulative mutations.
Taken together, these results suggest that trade-offs arise as levels of resistance escalate in the arms race with toxic newts. Accumulated mutations in the snakes beneficially confer toxin resistance, but also disrupt sodium channel function in muscle tissue, which ultimately causes reduced crawl speed. Our study is one of the first to link a few individual mutations in a conserved gene to broad trade-offs in performance at the level of the organism. These trade-offs are likely important in structuring population variation in snake resistance along the Pacific Coast, including at our field sites. Toxin-resistant snakes with a slow crawl speed may have lower survival if they are unable to quickly escape their own predators, like birds or mammals. This could explain why toxin resistance appears to be favored by selection in some populations, like Sonoma County, but disfavored in others, like Mendocino.
Mike Hague is a graduate student in the Brodie Lab, University of Virginia. The full study is freely available to read and download from Evolution Letters.