In the rigidly structured societies of bees, reproductive roles are strictly defined: queens bear the next generation, while workers support the colony’s survival through their labor. Ordinarily, the path to becoming a queen is determined by superior nutrition – queens are larger than workers and hence potential queens require more or better-quality food and are raised in specially designated royal cells. As a result, only just enough queens are reared to allow one to occasionally head a new daughter swarm. In honeybees, this leads to only 1 in 10,000 female larvae being reared as a queen.
An entirely different pattern is seen in roughly 70 stingless bee species of the genus Melipona, native to South America. Here, queens and workers, though morphologically distinct, are similar in size, and are reared in identically-sized closed cells. This allows larvae the autonomy to determine their own caste. However, as each female is genetically most related to its own offspring, evolutionary theory predicts that females should benefit from strategically biasing their development towards the queen pathway. Indeed, the production of queens in these species far exceeds the needs of the colony, with approximately 10% of all females developing as queens, in stark contrast to the pattern seen in other bee species. Most of these excess queens serve no useful purpose and are quickly executed by workers soon after emergence, leading to the loss of about 10% of the colony’s resources. This paradoxical outcome is a classic “tragedy of the commons” scenario, driven by a clash between individual and collective interests.
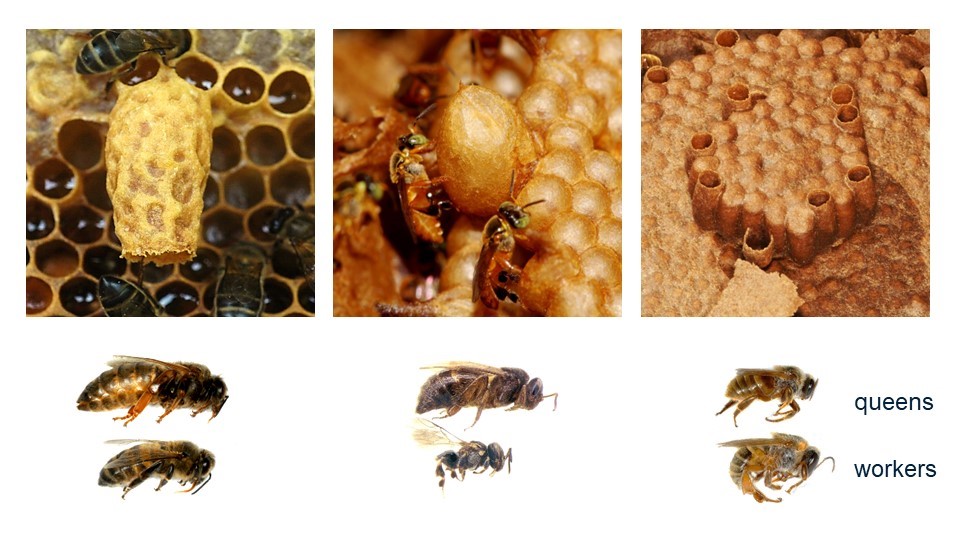
Depicted species and image credits: left: Apis mellifera queen cell from Wikimedia Commons (top) and queen and worker by Tom Wenseleers (bottom), middle: Tetragonisca angustula queen cell (top) by Cristiano Menezes (specieslink.net/col/FCM/) and Nannotrigona melanocera queen and worker (bottom) by João M.F. Camargo, right: Melipona bicolor comb containing both queens and workers (top) and a Melipona beecheii queen and worker (bottom), both by Tom Wenseleers.
In our study, we aimed to use an evolutionary model to pinpoint why precisely 1 in 10 females develop as queens in these bees and whether this rate matches the optimal strategy from an individual standpoint. This concept, known in economics as a Nash equilibrium, is referred to by evolutionary biologists as an “unbeatable” or “evolutionarily stable” strategy. While prior attempts to quantify this optimum could explain the overproduction of queens in Melipona, they did not manage to closely match the empirical data. To allow for a better fit, we formulated a fully dynamic model, in which we calculated what impact a given level of queen overproduction would have on colony growth and reproduction. Subsequently, we calculated at what level of queen overproduction the individual benefit of developing as a queen would balance with the indirect genetic or “inclusive fitness” cost caused by having a less productive colony. The result was remarkable: this new model resulted in a near-exact fit to actual empirical data derived from over 20 species. Developing as a queen with a probability of 1 in 10 turned out to be the unbeatable strategy. Examples where biological traits can be predicted with such accuracy are rare. Previously, the best such examples have come from studies on sex ratios, which explain, for example, why most birds and mammals produce offspring in an equal male to female ratio.
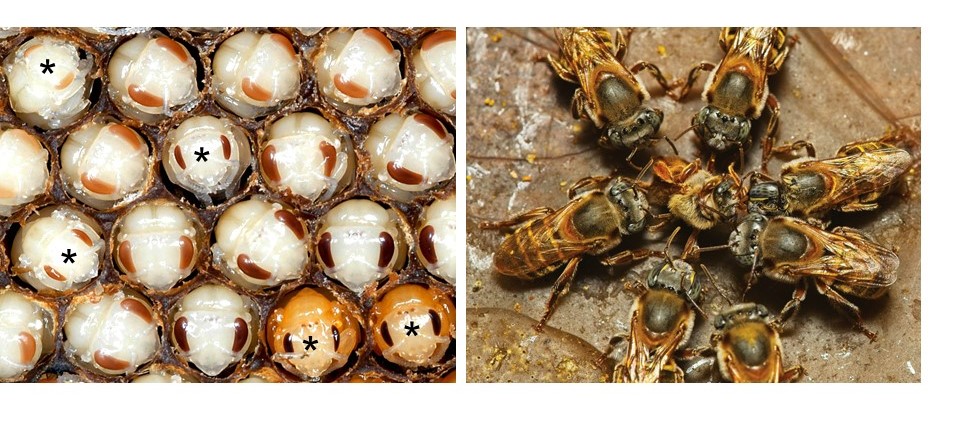
Depicted species and photo credits: left: Melipona subnitida, photo by Tom Wenseleers; right: Melipona beecheii, photo by Jorge Ramirez Pech.
Our findings suggest that when costs and benefits can be modelled in sufficient detail, social evolution models can make remarkably accurate predictions, explaining even the most counterintuitive natural phenomena. We hope our methods will inspire further research on how the balance between cooperation and conflict is set in other biological systems.